NASA's Spitzer Finds Water Vapor On Hot, Alien Planet, ScienceDaily, July 11, 2007 ... A scorching-hot gas planet
[Left: Artist's impression of gas giant planet HD 189733b transiting its star HD 189733 A]
beyond our solar system is steaming up with water vapor, according to new observations from NASA's Spitzer Space Telescope. See also: ABC, BBC, Discovery News, EurekAlert!, New York Times, SPACE.com, Telegraph, The Age, The Australian. First, this is another great technical achievement and my criticism is only of the hype that usually accompanies such discoveries, in support of an Epicurean materialistic "plurality of inhabited worlds" worldview. The headline, "NASA's Spitzer Finds Water Vapor On Hot, Alien Planet" may be a sign that journalists and editors are starting to realise that in fact, "More than 200 extrasolar planets have been found so far, but none is like the Earth" (my emphasis)!
The planet, called HD 189733b, swelters as it zips closely around its star every two days or so. Astronomers had predicted that planets of this class, termed "hot Jupiters," would contain water vapor in their atmospheres. This was a fairly safe prediction in that it was already known that in our Galaxy, "water is the third most common molecule in the regions studied." But see below that a rocky planet having an atmosphere, let alone it containing water vapour (or liquid water on its surface), is not automatic, and indeed my well be the exception.
Yet finding solid evidence for this has been slippery. These latest data are the most convincing yet that hot Jupiters are "wet." While "hot Jupiters" may very well have water vapour (i.e. superheated steam) in their atmosphere because presumably it cannot escape due to such massive planets' strong gravity, logically just finding it in one and not yet in any of the other ~100 is not "convincing" evidence that all (or even most) are "wet".
"We're thrilled to have identified clear signs of water on a planet that is trillions of miles away," said Giovanna Tinetti, a European Space Agency fellow at the Institute d'Astrophysique de Paris in France." Tinetti is lead author of a paper on HD 189733b appearing today in Nature. Although water is an essential ingredient to life as we know it, wet hot Jupiters are not likely to harbor any creatures. Water is only one of many "essential ingredient to life as we know it." Christian astronomer Hugh Ross points out there are "more than a hundred different requirements ... for life to exist on any given planet."
But even if all the requirements for life (i.e. all the ingredients of a bacterium) were together at the same time and place (which itself would be a miracle!), as origin of life researchers Morowitz and Shapiro have shown, the probability that the "essential ingredient to life as we know it" would spontaneously assemble is of the order of "1 chance in 10100,000,000,000" which "is so large that it reduces all considerations of time and space to nothingness ... the time until the black holes evaporate and the space to the ends of the universe would make no difference at all" (my emphasis):
"We are now ready to handle the chances for the spontaneous generation of a bacterium. ... For our purposes, we will want to overestimate and select the largest number of random trials that might have been attempted on the early earth, as the actual number would be very difficult to determine. We need to know two items, the length of time needed for a single trial and the number of trials that can take place simultaneously. Under the most favorable conditions, an E. coli colony can double in about twenty minutes. In other words, it takes twenty minutes for a bacterium to assemble a replica of itself from simple chemicals. It is unlikely that a bacterium would come together more quickly by random processes. Let us presume, however, that a simpler bacterium than E. coli is involved, and estimate one minute as the time for a trial. If we accept the evidence of the fossils and the usual age cited for the solar system, then a maximum of 1 billion years, or 5 x 1014 minutes, was available for the origin of life on earth. What about available space? As a maximum estimate, we can assume that the entire earth was covered by an ocean 10 kilometers deep, which was available for experiments. Further, we will allow that space to be divided into small compartments (1 micrometer on each side) of bacterial size. We would then have 5 times 1036 separate reaction flasks. If a separate try was made in each flask every minute for 1 billion years, we would have 2.5 times 1051 tries available. .... As a rough rule, we will consider that an event becomes probable when the number of trials available is of the same order of magnitude ... as the adverse odds on a single trial. ... We cannot compute these odds precisely, but approximations will serve our purposes quite well. ... A more realistic estimate has been made by Harold Morowitz, a Yale University physicist [Morowitz, H.J., "Energy Flow in Biology," Academic Press: New York NY, 1968, pp.5-12]. He has calculated the odds for the following case: Suppose we were to heat up a large batch of bacteria in a sealed container to several thousand degrees, so that every chemical bond within them was broken .... We then cooled this mixture slowly, in order to allow the atoms to form new bonds, until everything came to equilibrium. In this state, the most stable chemicals (those with the least energy) would dominate the mixture, while those with higher energy would be present to a lesser extent, in accordance with the laws of statistics. Morowitz asks, what fraction of the final product will consist of living bacteria? Or in other words, if a single bacterium was used to start the experiment (ensuring that the appropriate atoms, in proper amounts, were present), what would be the chances that a living bacterium would result at the end? The answer computed by Morowitz reduces the odds of Hoyle [1 in 1040,000] to utter insignificance: 1 chance in 10100,000,000,000.... This number is so large that to write it in conventional form we would require several hundred thousand blank books. We would enter `1' on the first page of the first book, and then fill it, and the remainder of the books, with zeros. ... The Skeptic will want to rewrite Professor Wald's conclusion: Improbability is in fact the villain of the plot. The improbability involved in generating even one bacterium is so large that it reduces all considerations of time and space to nothingness. Given such odds, the time until the black holes evaporate and the space to the ends of the universe would make no difference at all. If we were to wait, we would truly be waiting for a miracle." (Shapiro, R., "Origins: A Skeptic's Guide to the Creation of Life on Earth," Summit: New York NY, 1986, pp.125-128).
See also `tagline' quote below from Morowitz' book (minus most of the math symbols) that, to summarise:
"When we encounter such small numbers as" 10-10^11 = 10-100000000000 "no amount of ... arguing about the age of the universe or the size of the system can suffice to make it plausible that such a fluctuation would have occurred ... a number of authors on the origin of life have missed the significance of vanishingly small probabilities. They have assumed that the final probability will be reasonably large by virtue of the size and age of the system. ... this is not so: calculable values of the probability of spontaneous origin are so low that the final probabilities are still vanishingly small" (my emphasis)!
That is because, as Princeton Professor of Biology Harold F. Blum pointed out ~45 years ago, even if all "the proper molecular compounds" were to occur together, "whether on the earth... or elsewhere" then the problem would be to assemble them into a "living machine" in the same way that "an automobile" would not "spring spontaneously from a mixture of all the chemical species from which it is composed" (my emphasis):
"The living machine is clearly not just a mixture of chemicals, yet there seems to be widespread belief that, once the proper molecular compounds were there, life would appear, whether on the earth, on Mars, or elsewhere in the universe. This no more follows, I may point out at the risk of being thought overly facetious, than that an automobile, 1962 model, might spring spontaneously from a mixture of all the chemical species from which it is composed." (Blum, H.F., "Time's Arrow and Evolution," [1951], Harper Torchbooks: New York NY, 1962, p.178G)
So this entire field of astrobiology is based on a collective self-delusion (2Thess. 2:11) that if a truly Earth-like exoplanet is eventually discovered (and none have been yet), that will somehow show that life is common in the Universe and therefore `we are not alone'. It would show no such thing. They would still have to show what that have not been able to show in over a half-century of laboratory experiments, how the "essential ingredient to life as we know it" could, in the absence of intelligent design: 1) all occur together at the same time, same place and in the correct proportions and concentrations; and 2) how they could then self-assemble into a "living machine"!
Previous measurements from Spitzer indicate that HD 189733b is a fiery 1,000 Kelvin (1,340 degrees Fahrenheit) on average. And presumably all such "hot Jupiters" are "not likely to harbor any creatures." So if "hot Jupiters" turn out to be the majority of exoplanets (and not just the majority discovered to date) then the odds would become that much more unfavourable that life existed elsewhere in the Universe.
Ultimately, astronomers hope to use instruments like those on Spitzer to find water on rocky, habitable planets like Earth. The problem is that at those vast distances ("HD 189733b is located 63 light-years away") astronomers can detect only giant planets, like "`hot Jupiters,' which orbit their stars from a distance closer than Mercury is to our sun" that is "at a distance that is 30 times closer than that between the Earth and the sun." The huge difference in size between Jupiter-like and Earth-
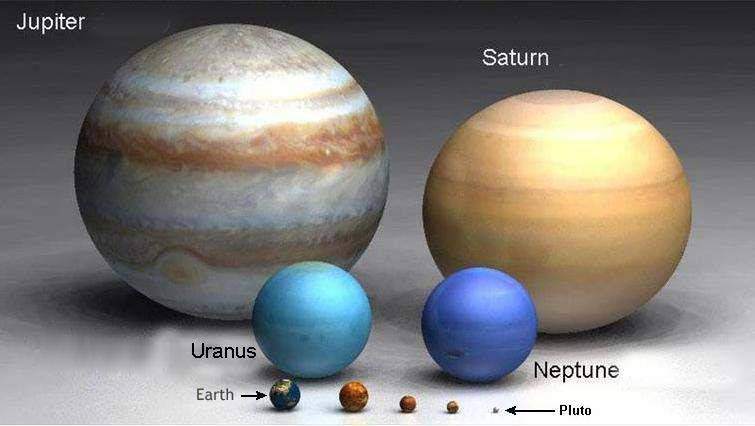
[Above: The size of Earth compared to Jupiter, etc, "The Size Of Our World"]
like planets, coupled with the latters' much greater distance from their star, means that it may well be that astronomers will never be able to detect whether "rocky, habitable planets like Earth" are even there, let alone whether they have "water" on them.
"Finding water on this planet implies that other planets in the universe, possibly even rocky ones, could also have water," said co-author Sean Carey ... This is fallacious to extrapolate from a sample of one. It is also misleading (deliberately so since he should know better). Having an atmosphere in the first place, and then for it to contain water vapour, is not automatic for "`terrestrial' planets or moons-those with solid bodies," since even in our solar system not all the "rocky" planets (i.e. not Mercury, Venus or Mars have atmospheric or liquid surface water," because "It turns out that getting an atmosphere-and holding on to it-really comes down to how big and how close to the sun you are ... or ... how close you are to a really big planet":
"Saturn's moon Titan belongs to a very select club within the solar system. It is one of only four `terrestrial' planets or moons-those with solid bodies, as opposed to those made largely of gas, like Jupiter and Saturn-that has a substantial atmosphere. The other three that wear blankets of gas are Venus, Mars, and our own Earth. Why just these four? Why not also, say, Mercury, Jupiter's biggest moons, our moon? How did those lucky four come by their atmospheres? It turns out that getting an atmosphere-and holding on to it-really comes down to how big and how close to the sun you are (or, for Titan, how close you are to a really big planet). ... The story of planetary atmospheres begins back at the beginning of our solar system, when the planets were forming. During that period, the so-called inner planets-Mercury, Venus, Earth, and Mars ... had sufficient gravity to draw these two gaseous elements in from the solar nebula, the vast cloud of gas and dust that surrounded the sun early in the solar system's history. In that primordial time, the sun was not very bright and thus not very hot, and this allowed the four inner planets to hold onto those atmospheres. ... This is where the how-close-you-are-to-the-sun part comes in. On Earth, all that water vapor belched out of volcanoes condensed in the young atmosphere into liquid water, then fell to the surface as rain. Over eons, this formed the oceans. Most of the CO2, meanwhile, became incorporated into the seas and into sedimentary rocks. ... As for Mars, its secondary atmosphere had two strikes against it from the start: the planet's size (too small) and its distance from the sun (too far). In its first 500 million years or so, the Red Planet had a warm atmosphere and liquid-water oceans, just like Earth. But Mars is so small that its internal heat engine burned out early on, and it is so far away from the sun that all the water vapor that its once-active volcanoes had erupted eventually froze out of the atmosphere, becoming trapped beneath the surface as ice. .... Mars still has an atmosphere, but its pressure is 100 times less than Earth's and it's almost entirely composed of CO2-about the last thing we'd want to breathe. Venus has roughly the same concentration of CO2 as Mars, yet its atmosphere went in precisely the opposite direction. Size wasn't an issue: Venus has about the same mass as Earth so is plenty hot within. But distance from the sun has made all the difference. Venus is near enough to our star that all the water vapor released from its volcanoes burned off long ago, and without liquid water, the planet could not form oceans that could absorb the CO2. " (Tyson, P., "How to Get an Atmosphere," NOVA, March 2006)
The new findings are part of a brand new field of science investigating the climate on exoplanets, or planets outside our solar system. Such faraway planets cannot be seen directly; however, in the past few years, astronomers have begun to glean information about their atmospheres by observing a subset of hot Jupiters that transit, or pass in front of, their stars as seen from Earth. So it may be that astronomers will not be able to detect the atmospheres, let alone whether they contain water, even for all "hot Jupiters," but only those that transit their star in a line of sight with Earth?
Earlier this year, Spitzer became the first telescope to analyze, or break apart, the light from two transiting hot Jupiters, HD 189733b and HD 209458b. One of its instruments, called a spectrometer, observed the planets as they dipped behind their stars in what is called the secondary eclipse. This led to the first-ever "fingerprint," or spectrum, of an exoplanet's light. Yet, the results came up `dry,' probably because the structure of these planets' atmospheres makes finding water with this method difficult. Later, a team of astronomers found hints of water in HD 209458b by analyzing visible-light data taken by NASA's Hubble Space Telescope. The Hubble data were captured as the planet crossed in front of the star, an event called the primary eclipse. Now, Tinetti and her team have captured the best evidence yet for wet, hot Jupiters by watching HD 189733b's primary eclipse in infrared light with Spitzer. In this method, changes in infrared light from the star are measured as the planet slips by, filtering starlight through its outer atmosphere. The astronomers observed the eclipse with Spitzer's infrared array camera at three different infrared wavelengths and noticed that for each wavelength a different amount of light was absorbed by the planet. The pattern by which this absorption varies with wavelength matches that created by water. "Water is the only molecule that can explain that behavior," said Tinetti. "Observing primary eclipses in infrared light is the best way to search for this molecule in exoplanets." Again, this is a great technical achievement to detect water vapour at such a vast distance, i.e. "63 light-years away" (see below).
The water on HD 189733b is too hot to condense into clouds; however, previous observations of the planet from Spitzer and other ground and space-based telescopes suggest that it might have dry clouds, along with high winds and a hot, sun-facing side that is warmer than its dark side. HD 189733b is located 63 light-years away in the constellation Vulpecula. .... It doesn't say how much water (i.e. steam) there was, just that there were detectable "water ... molecules." Presumably at "a fiery 1,000 Kelvin (1,340 degrees Fahrenheit) on average" all the water this planet has would be existing as superheated steam in its atmosphere and the total quantity of water could still be comparatively small?
Stephen E. Jones, BSc. (Biol).
Blogs: CreationEvolutionDesign & TheShroudofTurin
"At equilibrium, what is the probability that this system will, in fact, be a living cell? ... Suppose we were to grow a very large batch of cells of a bacterium such as Escherichia coli. We then centrifuge the cells into a tightly packed pellet of volume V containing N cells; transfer the pellet into a container of fixed volume V, and raise the temperature to some very high value (on the order of 10,000°C) so as to destroy any traces of the original chemical state of the system. Now slowly cool the system to 300°C and allow it to age indefinitely at this temperature. ... At this point, we can place an upper bound on pL by applying our knowledge of the chemical bonds found in actual living systems compared to the bonds found in the normal equilibrium state. ... From a thermodynamic argument, it is possible to compute the difference between the heat of formation of a group of biochemical compounds and the heat of formation of the lowest energy state possible for a system of the same atomic composition, volume, and temperature; hence, on the basis of gross molecular composition, we can estimate the energy difference between biological systems and their corresponding equilibrium systems. ... we shall set up a model system that will permit the calculation of pLmax. For the moment, we may anticipate the results of that calculation which shows that pLmax is the order of magnitude of 10-10^11 for a typical bacterial cell. The reason for pLmax having such an infinitesimally small value is that a living cell represents a configuration showing a very large amount of energy as configurational or electronic bond energy relative to the amount of thermal energy when compared with the equivalent equilibrium system. The living state has a very unlikely distribution of covalent bonds compared with the equivalent equilibrium state either at the same total energy or at the same temperature. ... Next, let us return to the number 10-10^11 which requires some discussion as many people are not accustomed to dealing with such infinitesimally small numbers. The number may be written 10-100000000000 or as a decimal; it may be written as a decimal point followed by 99,999,999,999 zeros followed by a 1. The number occurred as the maximum probability of a given ensemble member being alive. Suppose we have an ensemble of W members and we sample it at the rate of X times per second for Y seconds. We may then ask the question: what will be the probability of a living member having occurred once? This will be pLWXY. To place our argument in the context of terrestrial biology, let us assume the maximum possible values of W, X, and Y for the surface of the earth. (a) Wmax = 10100. This is a very generous estimate of the number of atoms in the universe and must, therefore, represent an outside upper limit to the numbers of members of the ensemble. (b) Xmax = 1016 sec-1. Since we are dealing with atomic processes, sampling times cannot be appreciably shorter than times for atomic processes which have a lower limit of about 10-16 sec. (c) Ymax = 1011 sec. Assume that the age of the universe is ten billion years, which appears to be an upper limit from current estimates. Utilizing these estimates, WmaxXmaxYmax is equal to 10134. Note, however, that pLmax ... ~ 10-10^11 ... When we encounter such small numbers as pLmax, no amount of ordinary manipulation or arguing about the age of the universe or the size of the system can suffice to make it plausible that such a fluctuation would have occurred in an equilibrium system. It is always possible to argue that any unique event would have occurred. This is outside the range of probabilistic considerations, and really, outside of science. ... We may sum up by stating that on energy considerations alone, the possibility of a living cell occurring in an equilibrium ensemble is vanishingly small. It is important to reiterate this point as a number of authors on the origin of life have missed the significance of vanishingly small probabilities. They have assumed that the final probability will be reasonably large by virtue of the size and age of the system. The previous paragraph shows that this is not so: calculable values of the probability of spontaneous origin are so low that the final probabilities are still vanishingly small." (Morowitz, H.J., "Energy Flow in Biology: Biological Organization as a Problem in Thermal Physics," [1968], Academic Press: New York NY, Second printing, 1969, pp.5-7,11-12)
No comments:
Post a Comment